Saving... Please wait..!
Poster Sessions
Delegates will be able to access and enjoy two forms of technical educational content at the event, along with the main technical program, technical posters will be displayed and presented on the exhibition floor. The technical posters will cover a wide range of technical topics in the hydrogen industry and be presented by the authors during specific scheduled times throughout the two days at the Canadian Hydrogen Convention.
Access to the poster session on the exhibition floor is included with a visitor, strategic and technical conference passes.
Come and meet the authors of the posters at the designated times below throughout the two days of the exhibition and conference.
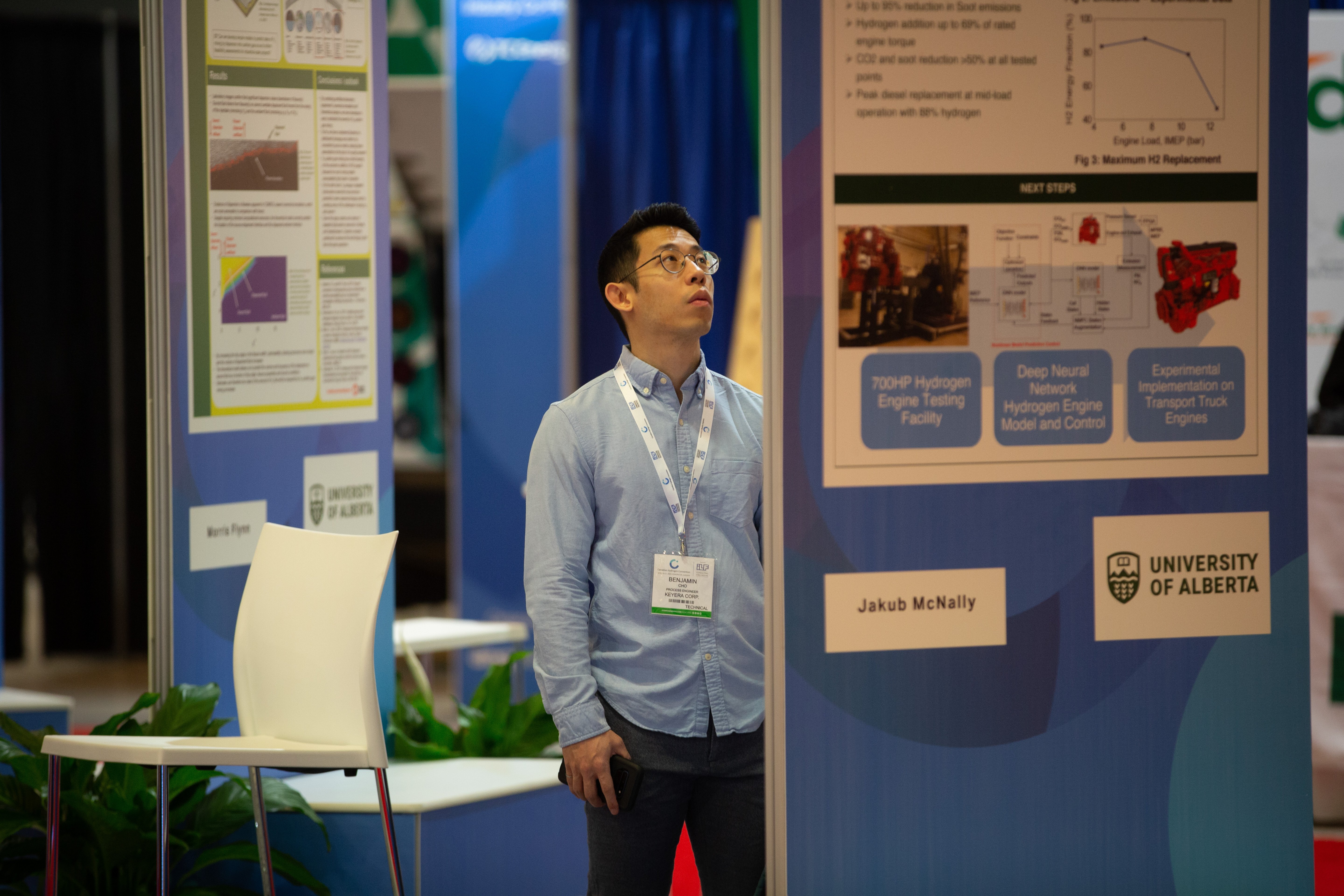

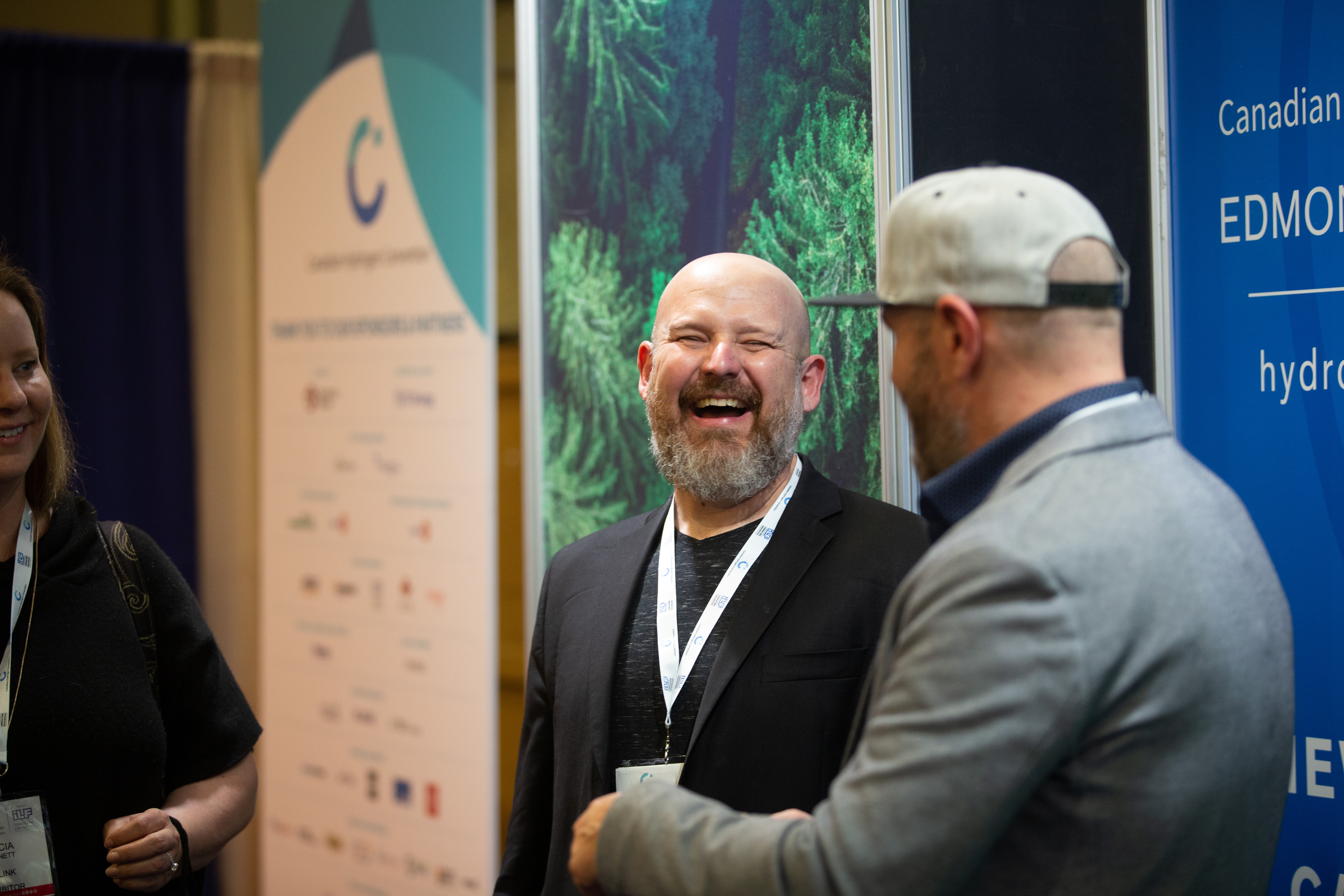
Location: Exhibition floor
Come and meet the authors of the posters at designated times throughout the two days of the exhibition and conference.
Tuesday
April 29, 2024
12:00 PM - 1:30 PM
2:30 PM - 3:00 PM
Wednesday
April 30, 2024
12:00 PM - 1:30 PM
2:30 PM - 3:00 PM
Beware of email scams
DMG Events has been informed from several exhibitors are receiving scam emails . Please be aware these offers are fraudulent. These scammers do not have any relationship with DMG Events.In accordance with GDPR, CASL and other jurisdictional data privacy regulations, DMG Events will never sell your data to any third party organisations. Please see our Privacy Policy for more information regarding how DMG Events will process and store your information.